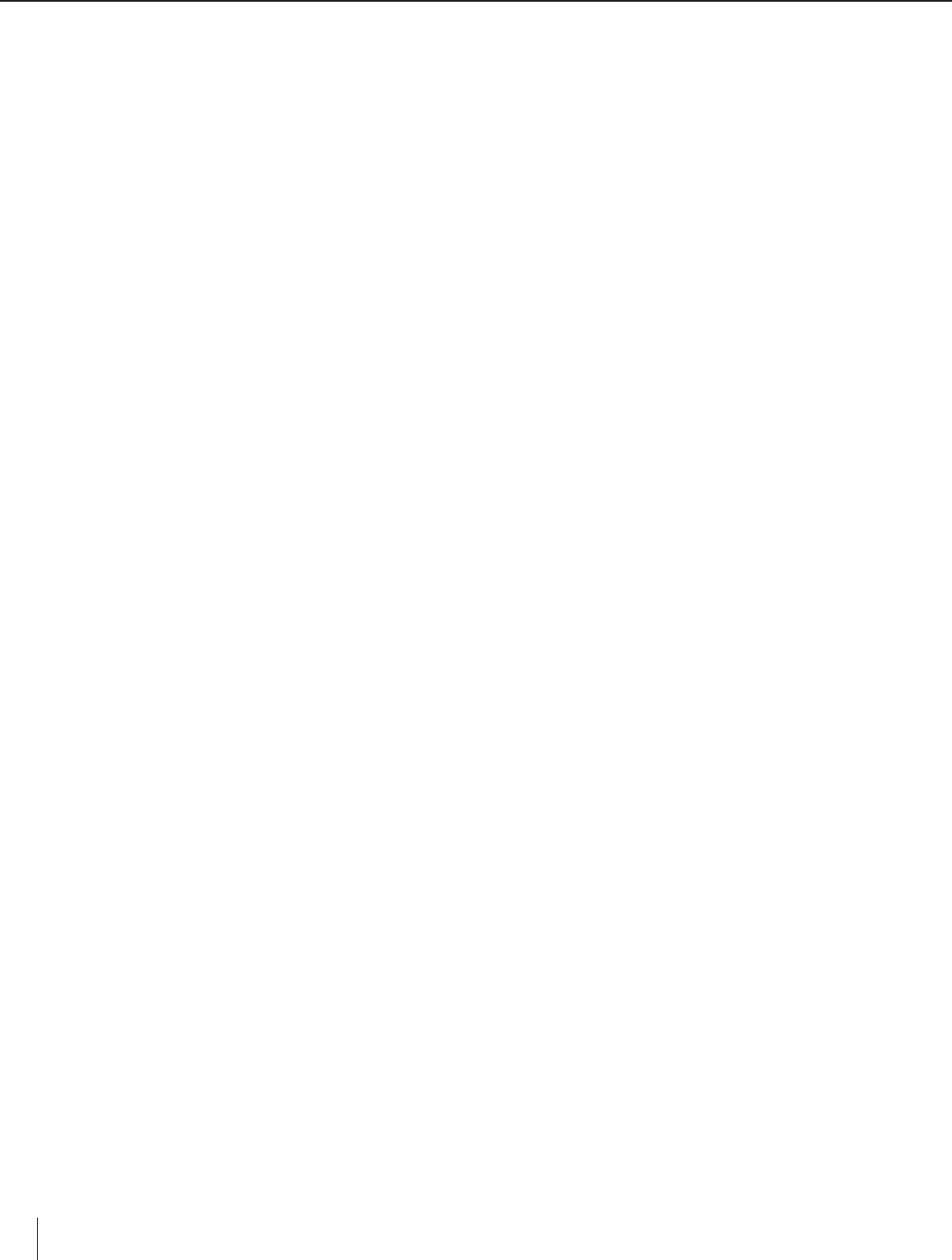
Petri Dish Electrolysis continued
3
© 2016 Flinn Scientific, Inc. All Rights Reserved.
17. Pour the solution from the Petri dish into a waste beaker and rinse well with distilled water.
18. Place the clean Petri dish on the overhead projector stage and add about 20 mL of 1 M tin(II) chloride solution.
19. Connect one paper clip to each alligator clip lead on the battery cap and attach the paper clips to opposite sides of the Petri
dish.
20. Attach the battery cap with alligator clip leads to the 9-V battery and observe the changes at the anode and the cathode as
electrolysis proceeds. A milky-white cloudiness appears at the anode due to tin(IV) chloride and beautiful metallic tin(0)
crystals form at the cathode.
21. Allow the reaction to continue for 1–2 minutes and observe the pattern of crystal growth. Tin crystals grow in a feather-like
(fractal) pattern, producing a beautiful “crystal tree.”
22. Gently remove the alligator clip leads from the paper clips and carefully switch the polarity of the electrodes. Attach the
alligator clip from the negative battery post to the previous anode (left-hand side above) and the alligator clip from the
positive battery post the previous cathode (the right-hand side above.)
23. Observe the changes at the new anode and the new cathode. The reactions are reversed—new tin crystals grow from the
previous anode and the tin crystals at the previous cathode dissolve into solution.
24. Disconnect the battery cap from the battery to stop the reaction.
25. Carefully decant the solution from the Petri dish into a clean waste beaker (avoid pouring the tin crystals). The tin crystals
may be poured onto several layers of paper towels.
26. The electrolysis products may include dilute halogen solutions (chlorine, bromine, and iodine). Working in the hood,
carefully pour the contents of the Petri dish into a waste beaker containing sodium thiosulfate solution. Sodium thiosulfate
will reduce the halogen waste products. Allow the beaker to stand in the hood overnight.
Disposal
Please consult your current Flinn Scientific Catalog/Reference Manual for general guidelines and specific procedures govern-
ing the disposal of laboratory waste. Electrolysis of potassium iodide generates iodine. The waste solution may reduced with
sodium thiosulfate according to Flinn Suggested Disposal Method #12a. The acidic tin chloride waste solution may be neutral-
ized with base according to Flinn Suggested Disposal Method #24b.
Tips
• Electrolysis of sodium chloride generates chlorine gas at the anode. Based on their standard reduction potentials,
oxidation of chloride ion to chlorine (E
o
= –1.36 v) should be less favorable than oxidation of water to oxygen
(E
o
= –1.23 V). However, there is a significant overvoltage for oxidation of water, and oxidation of chloride competes
with oxidation of water under typical electrolysis conditions. Although the cause of the overvoltage is poorly understood,
it is generally believed to be due to a kinetically slow reaction at the anode.
• Potassium iodide solution is light- and air-sensitive. Prepare the solution fresh within two week of its anticipated use and
store the solution in a dark bottle.
• Electrolysis of salt solutions takes place at concentrations greater than about 0.2 M. We recommend using 0.5 M
solutions for potassium iodide and sodium sulfate—electrolysis is very rapid and the color changes are more obvious than
in 0.2 M solutions.
• A black coating forms on the paper clip electrodes when the paper clips are placed in the tin(II) chloride solution. This is
due to a spontaneous single replacement reaction between the metal in the paper clips and tin(II) ions. The black coating
will not interfere with the electrolysis reaction.
• Electrolysis is a challenging topic for students. The demonstration provides a great critical-thinking (inductive
reasoning) exercise for students to identify the products of the separate half-reactions based on the observations at each
electrode. Write down all of the possible oxidation and reduction half-reactions for each salt solution on the board, and
ask students to interpret the evidence and determine the actual products.